The History of Batten disease
Four children with probable Juvenile Batten disease were first described by Otto Christian Stengel, MD, in 1826.1 He noted that four siblings developed normally until each began to lose their sight around age 6 leading to blindness and followed by progressive mental decline, loss of speech, seizures and death. The disease, however, is named after Frederick Batten, MA, MD, FRCP, when he reported almost 100 years later on two sisters with vision loss, neurocognitive decline and motor problems separating it from recently discovered Tay-Sachs disease and macular degeneration.2,3
Juvenile Batten disease
Juvenile Batten disease is one of a group of disorders known as neuronal ceroid lipofuscinoses (NCLs). Over 400 different errors (mutations) in 13 segments of DNA (genes) have been attributed to various forms of Batten, which primarily differ from one another by when symptoms first appear.4,5 These disorders all affect the nervous system with increasing seizures, movement disorders, altered thought processes, and cognitive decline. Childhood NCLs also include vision loss but adult onset Batten typically does not.6,7 Although Batten disease was originally used to describe only the juvenile form, the term “Batten disease” is widely used to refer to all forms of NCL. Click here for more information.
The CLN3 gene
Juvenile Batten disease results from mutations (mistakes) in the CLN3 gene (blueprint) responsible for making CLN3 protein. More than 60 different mutations in the CLN3 gene have been shown to cause juvenile Batten disease (Mole, 2011). However, most children with the disease are missing the same string of 966 DNA building blocks from the CLN3 gene; this mutation is known as the “1kb deletion.8” Click here for more information on the CLN3 gene and its protein.
Batten disease and other NCLs are linked to a buildup of substances called lipofuscins (lipopigments) in the body’s tissues. These lipopigments are made up of fats and proteins. Their name comes from the technical word “lipo,” which is short for lipid, or fat, and from the term “pigment,” used because they take on a greenish-yellow color when viewed under an ultraviolet light microscope. The lipopigments build up in brain cells as well as in skin, muscle, and many other tissues. Inside the cells, these pigments form deposits with distinctive shapes that can be seen under a more powerful electron microscope. CLN3 deposits look like fingerprints.9 Healthy lysosomes would normally dispose of these lipofuscins just as they recycle and get rid of other cellular wastes.
Many cells in the body contain CLN3 protein: skin cells (the cells that are biopsied for diagnosis), muscle cells, kidney cells, liver cells, etc. However, these cells do not die from having malformed or absent CLN3 protein, and it is unclear whether they are affected by all of that material that builds up. So why are neurons so harshly – and uniquely – affected?
Neurons have advanced communication skills. They transmit information through both chemical and electrical signals via specialized structures called axons and dendrites.10 We are not sure why neurons are selectively affected, but we know that these cells are particularly susceptible to damage when their recycling centers fail to work properly, which occurs in many neurodegenerative diseases like CLN3 disease, other neuronal ceroid lipofuscinoses (NCLs), and over two-thirds of the 50 lysosomal storage diseases.11 Although the genetic basis for many of these diseases is clear and some of the biochemistry of missing or affected proteins is well understood, the cellular mechanisms by which deficiencies in these proteins disrupt neuronal viability remain ambiguous. One analogy is to think of neurons as the Lamborghini of cells, capable of providing high performance but very delicate in design.
Where we are Today
It is difficult for anyone to grasp and to integrate the overwhelming complexity of a disease that affects the brain. Despite over 100 years of study, the only tools in a physician’s toolbox to treat CLN3 disease are palliative. Anti-epileptics are very effective for treating seizures. Anti-depressants are used widely for anxiety and other mood disorders. Many families report that physician-prescribed L-DOPA alleviates Parkinson-like movements.12 Unfortunately, these treatments have no effect on the progression of disease. Thankfully, the tide is turning. By incorporating findings in neurodegeneration, characteristics of CLN3 disease, and employing advanced technologies, academic investigators, clinician scientists, and pharmaceutical scientists are developing treatments with the potential to support health and block disease.
Overview of DNA, RNA, Protein and Cellular Function Medicines
To help you understand ongoing approaches to treatment, BBDF has organized the following CLN3 disease State of the Science report into therapeutic approaches from: 1) addressing the primary reason for – or genetic culprit of the disease (DNA), 2) the missing or damaged downstream RNA used as a template to make protein, 3) CLN3 protein, 4) cellular function affected by the loss of healthy CLN3 protein, and 5) cellular transplantation.

Figure 1: DNA, RNA, Protein
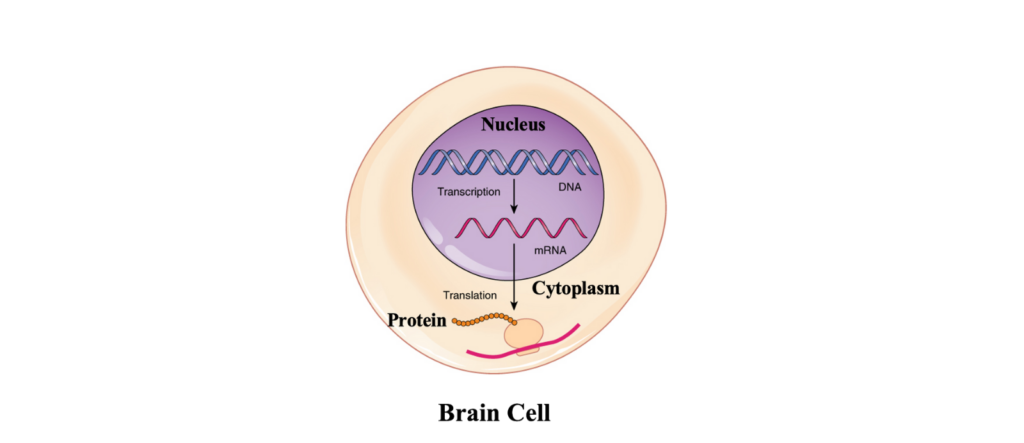
Figure 2: Transcription of CLN3 gene (DNA) to CLN3 RNA intermediate, Transport of CLN3 RNA out of Nucleus, Translation of CLN3 RNA into CLN3 Protein responsible for cellular function(s).
DNA as medicine
Academic and Pharmaceutical Scientists are working around the clock to create CLN3 gene therapy drugs using certain viruses. An adeno or lentivirus, modified so that it cannot cause disease, is used to “infect” cells with healthy CLN3 genes. Modified viruses can be given intravenously, traveling through the bloodstream and being taken up by cells throughout the body, or through intrathecal injection at the base of the spine, traveling through the cerebral spinal fluid to be taken up by cells in the brain and spinal cord.
Amicus
Amicus Therapeutics initiated a Phase 1/2, open-label (no placebo group), single dose AAV9 CLN3 gene therapy via intrathecal injection in December 2018. There are two Cohorts, one with a low dose and the second a high dose. The primary outcome for this trial is to evaluate safety. The co-primary objective of the study is to determine the efficacy of Amicus’ CLN3 gene therapy as measured by the Unified Batten Disease Rating Scale physical subscale. In May 2020, the USFDA granted Fast Track Designation to the CLN3 Batten disease gene therapy program. The Fast Track program facilitates the development and accelerates the review of new drugs for serious conditions, which have the potential to address unmet medical needs. A drug development program with Fast Track designation is afforded greater access to the FDA for the purpose of expediting its review and potential approval. Amicus plans to begin a second, interventional trial, for CLN3 disease in 2021.
Abeona
Abeona Therapeutics is in preclinical development to deliver single-dose, AAV-based gene therapy to CLN3 patients via intravenous injection. According to Abeona’s 2020 Annual Report, ABO-201 (scAAV9.CLN3) shows efficacy in mouse models of CLN3 disease following the delivery of a functioning copy of the CLN3 gene. Previous studies show reduced cellular waste buildup (lysosomal storage) and decreased inflammation (astrocyte/microglia activation) in the brain as well as improved motor function following treatment.13
As of December 2020, Abeona has not released a timeline to filing an Investigational New Drug application to initiate a clinical trial in CLN3 disease.
Retinal Gene Therapy
Presently, the academic community is working on retinal gene therapy. No specific therapeutic development plans have been announced from the pharmaceutical sector. However, the approval of Luxturna, which delivers a full healthy copy of the RPE65 gene directly to retinal cells using an AAV vector via subretinal injection in patients with Leber congenital amaurosis, a disease similar to CLN3 disease in its genetic underpinnings and progression, gives us hope. Published work by Robin Ali’s group at King’s College London demonstrating the potential to treat CLN3 blindness is an exciting development.
Currently, gene therapy is an area that exists predominantly in research laboratories and clinical trials. The first US approval of a gene therapy occurred in 2017and the pace is now picking up. For a current and complete list of FDA-approved gene therapies go to https://www.fda.gov/vaccines-blood-biologics/cellular-gene-therapy-products/approved-cellular-and-gene-therapy-products. This list is expected to continue growing as developments in viral vectors enhance our ability to target specific tissues, cross barriers like the blood brain barrier, and more fully penetrate treated tissues. Our ability to regulate the timing and quantities of gene product released will affect which diseases ultimately benefit from this technology. Virologists and immunologists are working on ways to deliver genes without patients having an immune or rejection response to the therapy. Studies suggest that an average of only 20% of treated organs take up the vector and its genetic cargo and that up to 40% of patients have some sort of rejection response to the treatment. Many researchers and clinicians believe that effective therapy for CLN3 disease will require a cocktail of medications and approaches including gene therapy.
Antisense Oligonucleotides
Michelle Hastings, PhD of Rosalind Franklin University of Medicine and Science is working with Ionis Pharmaceuticals using RNA to create a modified version of the CLN3 protein. When CLN3 gene DNA is mutated with the common ~1kb deletion, the resultant RNA intermediary contains a stop signal in the middle of its reading frame such that no normal, full-length CLN3 protein can be made from that RNA.5,14 Dr Hastings and her colleagues demonstrate that a single treatment of neonatal mice with CLN3 antisense oligonucleotide (ASO)-skipping exons 5-8 of 15 inhibits disease progression for over 1 year. Animal models of disease exhibit decreased accumulation of cellular waste in brain tissues, have fewer signs of chronic inflammation, and have improved motor coordination in comparison with untreated animals. Using a new hybrid animal model with genetic defects associated with Alzheimer’s and CLN3, CLN3 ASOs improved survivability by 186%. When tested in cultures of skin cells obtain from affected children, researchers discovered that the ASOs were able to produce the same result as in the animal models of disease. 15 IonisPharmaceuticals, together with Dr Hastings, is working to identify and optimize their top ASO for clinical trial development.
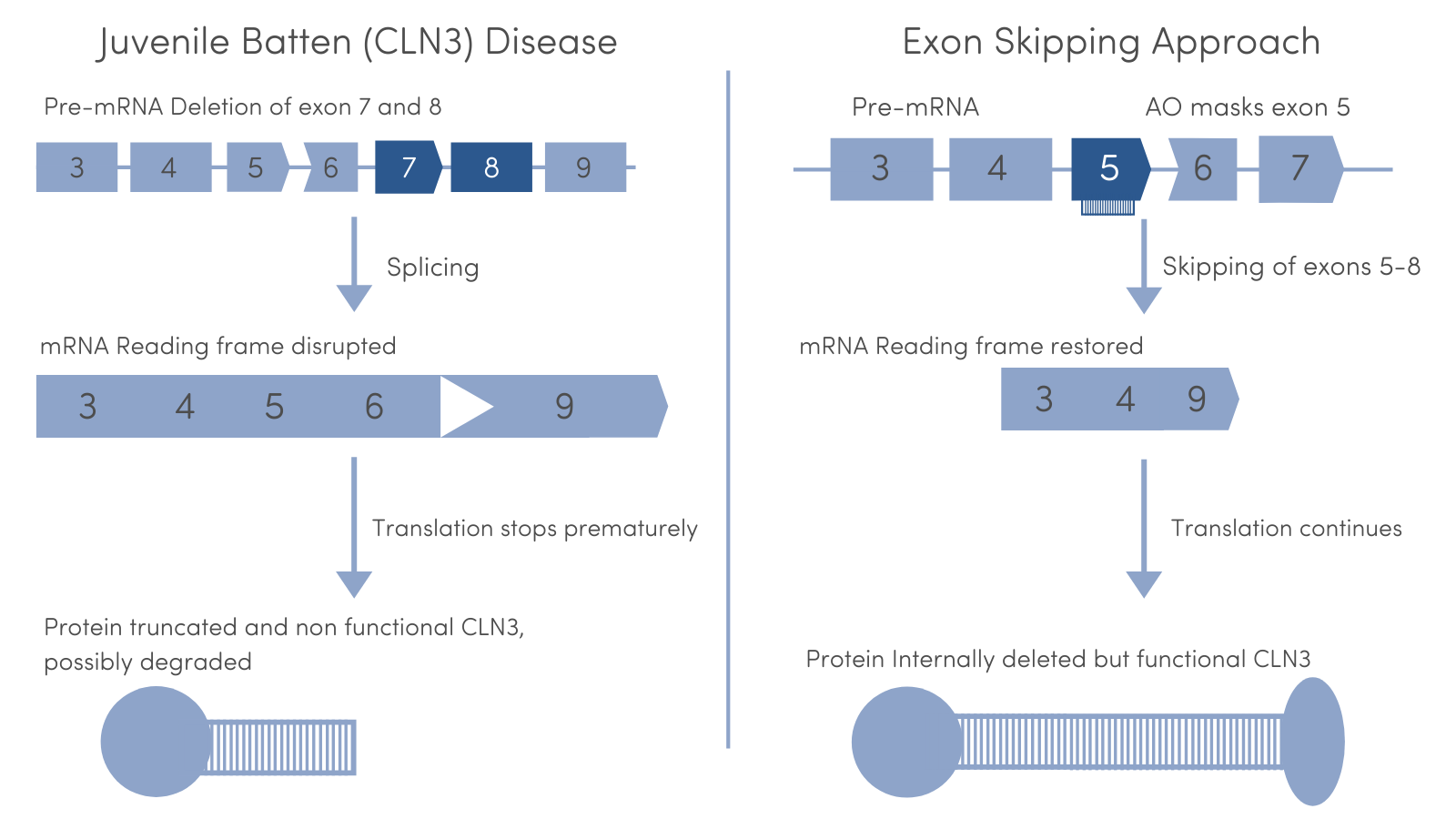
Figure 3: Antisense Oligonucleotide treatment
RNA as medicine
Normally, the alphabet-like code of DNA is “read” (transcribed) to make an intermediate molecule called ribonucleic acid (RNA). The code of this template is then translated into protein, the workhorses of our body. So, you can see how a mistake in one’s DNA results in a mistake in RNA and a problem with the protein. Some proteins have simple jobs, others more complex – provide structure, act as messengers, transport nutrients, or provide energy. Some proteins have more than one job and some exert control over other proteins such as when those proteins are made, how much, where, and under what circumstances so that brain cells do what they do when they need to.When targeting a genetic illness, like CLN3 disease, there are many ways to “fix” the problem – replace faulty gene or add new supportive genes using gene therapy, edit the RNA templates to deliver an otherwise absent protein or deliver normal healthy RNA directly.
Direct Delivery (Acturus and Moderna)
Delivering RNA directly to affected cells is a new category of medicine. Researchers start with the sequence of the missing protein to reverse-engineer the RNA that can be placed into cells. Once the RNA is in the cell, biology takes over and the cell makes, edits and transports the protein to its intracellular work sites. RNA delivery is especially attractive for treating CLN3 disease due to its transmembrane protein defect. Unlike the CLN2 form of Batten disease, for which there is an FDA-approved tripeptidyl peptidase 1 (TPP1) enzyme replacement therapy.16 CLN3 protein is a transmembrane protein and not a soluble enzymatic protein. Transmembrane proteins, cannot be delivered to the body in an IV solution, and cannot travel through the brain or between affected cells. CLN3 transmembrane protein sits inside the walls of the lysosome and other intracellular compartments.17 Introducing RNA into affected cells ensures that the natural machinery of the cell produces, processes and transports the CLN3 protein correctly to its worksite.
In 2019, the Cystic Fibrosis Foundation entered into a partnership with Acturus Therapeutics for $15 million to deliver normal cystic fibrosis transmembrane conductance regulator (CFTR) RNA to affected cells in the lung. The goal of the program is to produce an Investigational New Drug (IND) application for RNA therapy. Acturus, Moderna and many other companies are perfecting delivery vehicles for RNA to reach the Central Nervous System. BBDF is engaged in discussions with Moderna to capitalize on this technology for children with CLN3 disease as soon as possible.
Protein as medicine (Exosome delivery)
As mentioned above in the RNA-as-medicine section, CLN3 protein is not soluble so it cannot be directly inserted into the body much like enzyme replacement therapy approved by the FDA for the CLN2 form of Batten disease.16 However, Pharmaceutical companies are examining ways to transport difficult proteins like the CLN3 protein into the central nervous system.
One possible method for introducing normal, healthy CLN3 protein to an affected child is through exosomes. Exosomes are naturally-occurring membrane-bound vesicles located throughout the body that target, communicate, and carry cargo between cells. By binding to receptors on the cell surface of recipient cells, cargo-carrying exosomes fuse with the membrane of the recipient cell (like soap bubbles merging with one another) and deposit their cargo. Scientists have recently determined ways to isolate, sterilize, fill and deliver cargo-filled exosomes into the body. Designing CLN3-carrying exosomes could carry CLN3 RNA or CLN3 transmembrane protein to and through hard-to-reach brain tissues.18
CLN3 (dys)function as the drug target
As described above, efforts to repair CLN3 DNA and introduce CLN3 RNA and/or protein are underway across academic and pharmaceutical communities to address the root cause of CLN3 disease.
There is a fourth category of medicines – those that target missing or aberrant cellular functions. We do not know the exact function of CLN3 protein. However, we do know what happens when it is missing or deficient – Batten disease. Therefore, we can focus on what we know is going wrong to restore function and/or inhibit disease.
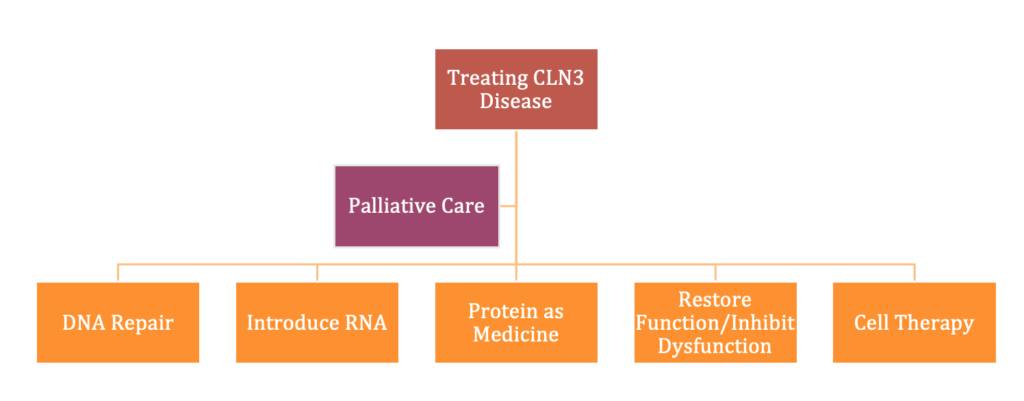
Figure 4: Medicinal Categories
Just like accident reconstructionists work backwards from multicar pileups to determine whether and to what degree excessive speed, or driver error contributed to an accident, CLN3 disease researchers work backwards from the cellular disaster they see under a microscope to determine which disease-initiated action happened first or which was the most devastating to which healthy functions. If one can pharmacologically stop disease-created event(s) from happening and/or help restore compromised cellular functions, cells remain healthy, the brain remains healthy and a child with CLN3 disease will survive and perhaps thrive. Children affected by CLN3 disease develop normally, even excelling in school.19 If we can tip the scales back towards health, we can change the lives of affected children and their families.
TFEB activation
Intense scrutiny of patient cells and animal models of CLN3 disease over the past 3 decades demonstrates that the loss of CLN3 protein impacts multiple cellular functions. The majority of dysfunction is in the lysosomal endosomal system, a series of cellular compartments responsible for internalizing waste and recycling parts of the cell during a process called “autophagy.” Autophagy is a self-degradative process that is important for housekeeping within cells removing misfolded or excessive proteins, clearing away damaged parts of a cell and recycling parts of the cell constantly renovating to maintain a high level of function and efficiency. Here is a short simplified video of the process https://youtu.be/Hqs1WzTwBEU:
Through the years, investigators have discovered defects at all levels of the autophagy process: the gathering of contents to be degraded (endocytosis), intracellular transportation, lysosomal enzyme content, the regulation of calcium and lipid levels, and the size, stability, positioning and pH of lysosomes.20-41 Children with CLN3 disease have lysosomes and they work. However, CLN3 disease-affected lysosome degradation pathways are inefficient and become overwhelmed leading to a toxic buildup of cellular waste.
In 2009, Sardiello, Ballabio and their colleagues observed that the expression of genes encoding multifunctional lysosomal proteins affected by the loss of CLN3 protein tend to be coordinated leading to the discovery that transcription factor EB or TFEB regulates over 400 intracellular proteins important for the coordinated production of new lysosomes, enzymes, transporters, membrane proteins, proteins responsible for lysosomal acidification, and lysosomal positioning.42-45 Researchers proposed that the pharmacological activation of TFEB could prompt cells with 100 inefficient lysosomes to make 1000, albeit inefficient, lysosomes and turn back the clock to a time when CLN3 disease-affected brain cells could handle their waste management needs.
BBDF-101
After 11 years and $31.5 million invested in overall research, the teams from BBDF, the Jan and Dan Duncan Neurological Research Institute, Cardiff University, Evotec, Charles River Laboratories, and along with key opinion leaders in Batten disease from University of Rochester, University Medical Center Hamburg-Eppendorf, and Texas Children’s Hospital are bringing a TFEB activator to clinical trial for children and young adults with CLN3 disease. In 2019, Theranexus, a publicly-traded European pharmaceutical company, committed the $20 million and expertise necessary to complete the clinical trial and commercialization of “BBDF-101.” To see the timeline of activity click here.
BBDF-101, a combination of intravenous trehalose and oral miglustat, activates TFEB leading to the creation of new lysosomes, enhanced lysosomal activity, clearance of cellular waste, reduced inflammation of the brain and rescuing brain cells and slowing the progression of CLN3 disease in animal models and patient cells.43,46,47
Trehalose is a naturally occurring disaccharide currently used as a mild sweetener and protein stabilizer in many pharmaceutical products such as eye drops and intravenous chemotherapy.48 Trehalose clears cellular waste accumulation in two ways by: 1) preventing accumulated protein waste from aggregating,49 and 2) activating TFEB leading to the coordinated production of new lysosomes.46,47 Miglustat, currently approved to treat Gaucher and Niemann Pick type C, supports the activity of Trehalose by preventing its degradation, specifically inhibiting the accumulation of a subtype of cellular waste (gangliosides) and inhibiting chronic inflammation within the brain.50 If you have a family member affected by CLN3 disease and would like to stay abreast of BBDF-101 clinical trial developments and other therapies you see here, please contact Mary Beth Kiser, president and CEO of BBDF, for information about our quarterly family calls. Additional efforts to find and to advance TFEB activating drugs into the clinic for CLN3 and other forms of pediatric and adult neurodegeneration are being pursued by Merck and Polaryx.
Calporta Therapeutics/Merck
In November 2019, Merck struck a deal to buy Calporta Therapeutics for up to $576 million in up-front and milestone payments. The purchase centers on transient receptor potential cation channel, mucolipin subfamily, member 1 (TRPML1) activators small enough to cross into the brain and activate TFEB (see Restore (Intracellular) Health through TFEB activation above). Early laboratory studies suggest Calporta’s TRPML1 agonists clear toxic waste build-up common in CLN3, ~60 other lysosomal storage diseases, and adult forms of neurodegenerative disease such as Parkinson’s, Alzheimer’s and amyolateral sclerosis (ALS).
Avalon Ventures and GlaxoSmithKline set up Calporta in 2015 as part of their collaborative pharmaceutical incubator. The work done by Calporta resulted in the creation and initial evaluation of multiple TRPML1 agonists. Merck will continue the evaluation and comparison of the agonists to reach the central nervous system and to activate TFEB with minimal side effects to the patient. To date, there are no public reports citing inclusion of CLN3 or other forms of NCL in Merck’s plans. However, BBDF is engaged in discussions with Merck to highlight the potential utility of a TRPML-1 therapeutic for children and young adults with CLN3 disease.
Polaryx
Fibric acid derivatives (fibrates; i.e. fenofibrate, bezafibrate, gemfibrozil) are a class of lipid metabolism medications most commonly known for their effectiveness in lowering serum triglycerides and very low density lipoprotein (VLDL; i.e. “bad”) cholesterol, and increasing high density lipoprotein (HDL; “good”) cholesterol.51 But that is not all fibrates do. Fibrates activate peroxisome proliferator activating receptor-α (PPAR-α), one of three PPARs which regulate gene expression by binding with retinoid X receptor as a heterodimeric partner to genes implicated in oxidative stress, energy homeostasis, mitochondrial fatty acid metabolism, glutamate metabolism, cholinergic/dopaminergic signaling and inflammation – all reported to be dysfunctional in CLN3 disease.29,31,34,40,43,52 Furthermore, fibrates have been shown to suppress programmed cell death (apoptosis); something that happens quite frequently in CLN3 disease.53
Interestingly, investigators at Rush University Medical Center suggest that fibrates activate TFEB when they reported that gemfibrozil treatment of CLN2 Batten disease models results in cellular clearance and improved survival over untreated animals leading the US FDA to grant Polaryx permission to conduct 12 month gemfibrozil treatment trials in children with CLN2 in January 2020.54 Approval to conduct a 12 month gemfibrozil treatment trial in CLN3 was given in April 2020 forms of Batten disease. 54 No trial initiation start date has been announced. Once enrollment begins, more information will be available on clinicaltrials.gov.
Unfortunately, there are a few concerns that dampen BBDF’s enthusiasm for the Polaryx program. According to the original proof of concept publication, the investigators did not measure known fibrate actions as a positive control for their study. Other laboratories have attempted to repeat the newfound results using cells from the same model, human induced pluripotent stem cells and patient lymphoblasts – all without success.56 Most importantly, gemfibrozil could have adverse effects on brain development due to suboptimal quantities of cholesterol necessary for forming connections between neurons for learning and memory and as a raw material for making Vitamin D, whose deficiency has been linked to dementia.57 While gemfibrozil may be safe for children with hyperlipidemia as evidenced by an 8-month study, children with hyperlipidemia have an intact blood brain barrier whereas children with CLN2 and CLN3 disease may not resulting in unsafe levels of gemfibrozil in the brain.55 Furthermore, eight months may not be long enough to determine adverse events associated with long-term use in CLN3 disease. For these reasons, BBDF does not currently support the Polaryx program yet remains open to the possibility of doing so should additional supportive safety and efficacy data came to light.
Sodium phenylbutyrate (SPB) and Tauroursodeoxycholic acid (TUDCA)
The pharmaceutical company Amylyx is spearheading the development of AMX0035, an oral combination therapy of repurposed drugs, for the treatment of neurodegenerative disease. Sodium phenylbutyrate (SPB) acts as a chemical chaperone to stabilize the conformation of folded protein and thus prevent proteins from aggregating in the endoplasmic reticulum (ER) prior to moving onto their cellular worksites.58 Tauroursodeoxycholic acid (TUDCA) inhibits cell death (apoptosis) mediated by dysfunction within cellular energy stores (mitochondria) via the buildup of reactive oxygen species.59 AMX0035 is a combination of the two.
While Amylyx has not released preclinical data that supports the conclusion that SPB and TUDCA together inhibits neurodegeneration more so than each individually, arguments in favor of the combination center around the fact that each compound has been shown to have a positive impact in animal models of neurodegenerative disease and each displays a different mechanism of action suggesting that together, their effects could be additive. SPB and TUDCA each reduce neuropathology, improve cell survival and ameliorate symptoms of Alzheimer’s, Parkinson’s, Huntington’s and amyolateral sclerosis (ALS).60-64
Amylyx conducted a 24-week, randomized, double-blind, placebo-controlled Phase II/III clinical trial to assess SPB/TUDCA’s safety, tolerability, and impact on disease progression resulting in statistically significant treatment benefits for people with ALS. Another trial in Alzheimer’s Disease shows early promise. BBDF is working with Batten researchers and Amylyx to design proof of concept studies in CLN3 disease that could lead to the development of AMX0035 for CLN3 disease. It is important to note that many researchers and clinician scientists believe that a “cocktail approach” of medicines targeting multiple intracellular drug targets at once, is our best bet for providing affected children with sustained health.
Phosphodiesterase‑4 Inhibitors
To better understand what is going wrong when brain cells are deprived of healthy CLN3 proteins, researchers at the University of Nebraska examined the effect of restoring the levels of cyclic adenosine monophosphate or cAMP in the brains of CLN3 mouse models.65 cAMP is a regulatory molecule involved in a wide variety of cellular activities important for learning and memory abilities which are severely compromised in CLN3 disease. If cAMP levels were compromised, this could help to explain why such devastating losses occur. Their work revealed that, similar to affected children, cAMP levels are significantly reduced in several brain regions of -CLN3 mouse models. To evaluate whether the normalization of cAMP levels can improve the health of CLN3-affected cells, investigators treated CLN3 mouse models with several phosphodiesterase-4 inhibitors rolipram, roflumilast and PF-06266047. Phosphodiesterase inhibitors block phosphodiesterase, which prevents the inactivation of cAMP effectively and increases increasing cAMP levels. Following subcutaneous injections of rolipram or oral applications of roflumilast or PF-06266047, lysosomal pathology was reduced, signs of inflammation were reduced, and glutamate transporter expression was normalized. Normalization of key molecules involved in glutamate clearance/detoxification could inhibit CLN3 disease-mediated excitotoxic neuronal death. In addition, targeting phosphodiesterase has been validated as an effective therapy for inflammatory conditions including chronic obstructive pulmonary disease (COPD), psoriasis, inflammatory bowel disease, and rheumatic arthritis and is in clinical trials for Alzheimer’s disease. BBDF is working to advance the development of PDE4 inhibitors for CLN3 disease.
Flupirtine
Flupirtine is a non-opioid has been approved for use in Europe for the last 36 years for pain management following surgery, trauma, and musculoskeletal pain. Additional properties include muscle relaxation, neuroprotection and anti-apoptosis (prevention of programmed cell death). Flupirtine has never been introduced in the United States for any indication. In 2002, Boustany and her colleagues showed that white blood cells (lymphocytes) taken from CLN3 patients exhibit less cell death in culture than untreated CLN3 lymphocytes and neurons derived from human teratocarcinomal line line (hNT) can be rescued from chemotherapy-induced cell death with flupirtine.66 In 2020, Boustany and her colleagues showed that the treatment of CLN3 knockout mice for 14 weeks inhibited cell death, motor dysfunction and cognitive impairments over untreated Batten mice.67 However, when researchers at the University of Rochester Batten Center examined health outcomes in children taking Flupirtine, they noted no obvious benefit despite parent-reported outcomes to the contrary.68 Therefore, results obtained in culture and in rodent models of disease may not translate into benefits for patients or may only benefit an as-yet-to-be-defined subset of patients. Note: In March 2018, the European Medicines Agency (EMA) removed flupirtine from pharmacy shelves because of the risk of serious liver injury. BBDF is currently unaware of countries where flupirtine is produced, sold or under preclinical or clinical investigation for use in CLN3 or any other disease.
Neuroinflammation
Insults incurred during disease progression generally fall under two definitions – those that are cell autonomous where underlying genetic defects in those cells lead to their own demise and outside forces such as extracellular plaques of beta amyloid in Alzheimer’s disease69 or inflammatory mediators in neurodegenerative diseases. In 2002, researchers reported their discovery of antibodies to glutamic acid decarboxylase (GAD), a neuronal enzyme involved in the synthesis of neurotransmitter gamma-aminobutyric acid (GABA) in mouse models and sera of patients with CLN3 disease suggesting the preferential or accelerated loss of GABAergic neurons.69
Mycophenolate mofetil (CellCept®)
Taken together with evidence of astrocytosis, microglial activation and immunosuppression studies in animal models, clinicians hypothesized that treating children with Cellcept™ (mycophenolate mofetil) could slow the progression of CLN3 disease.71 While the results of the randomized, double-blind, placebo-controlled, crossover study demonstrated that the drug was both safe and tolerable, the trial did not give any indication of potential efficacy.72 This may be due to the short length of the trial or the choice of immunomodulatory agent. Mycophenolate is commonly used to prevent organ rejection in children by depleting inosine-5’-monophosphate dehydrogenase which is required for T and B lymphocytes inhibiting their proliferation and suppressing cell-mediated immune responses and antibody formation. Studies in CLN3 disease have not demonstrated infiltration of T cells into the CNS and mycophenolate may have little to no effect on microglial activation or astrocytosis. BBDF does not invest in strategies that suppress the immune system. Instead, BBDF supports investigation into understanding CLN3-specific inflammation to carefully target only deleterious effects of the disease to without compromising one’s ability to fight off illness or infection.
Fingolimod and Teriflunomide
The potential therapeutic benefit of immunomodulatory compounds commonly prescribed to treat Multiple Sclerosis, fingolimod and teriflunomide, has been demonstrated in CLN3 mouse models. Oral administration of each reduced microgliosis, neuron loss, and resultant brain atrophy.73 Comprehensive studies are needed to determine the CLN3 disease-specific inflammatory cascade and apply the proper cocktail of MS-related and/or other anti-inflammatory agents without immunocompromising those with CLN3 disease. BBDF is following up on this line of inquiry.
Artificial Intelligence and Drug Discovery
We live in the age of “big data,” in which we have the capacity to collect huge sums of information too cumbersome for anyone to process alone, ultimately leaving no digital stone unturned. The entertainment industry harnesses big data to track our likes and dislikes to create new blockbuster films. Retailers follow emerging fashion trends and track inventory. BBDF’s work compiling all of the CLN3 DNA (gene), RNA and protein data from biological databases, repositories, and science and technology journals to create “The CLN3 Gene and Protein: What We Know” played a key role initial the development of a partnered program with Healx, a Cambridge (UK) technology company using artificial intelligence (AI) and bioinformatics to identify existing drugs or existing drug combinations that lessen the severity and/or inhibit CLN3 disease.17
Healx
Today, BBDF, Healx, NCL Stiftung and Haley’s Heroes are working together to generate and to collect high quality CLN3-specific information needed to populate Healx’s AI model. When complete, the structure- and function-based drug target, animal model, and clinical, and pharmaceutical information will be used to identify single- and multi-agent treatments for CLN3 disease. Healx’s technology has already been used successfully to identify treatments for Fragile X and over a dozen other diseases.
Cell (replacement) therapy
Stem cells, when directed by biological cues in the brain, offer the possibility to treat a myriad of intractable neurological conditions and disease. Preclinical research in animal models of some nervous system diseases shows that transplanted stem cells migrate to damaged areas leading to the partial restoration of function. Clinical trials are underway to test the safety and efficacy of stem cells from blood, bone marrow and umbilical cord blood to treat stroke, multiple sclerosis, spinal cord injury, Parkinson’s disease as well as CLN2, CLN3 and CLN5 disease. Follow up 0.5 to 5 years post-treatment did not demonstrate the inhibition of disease or other benefits suggesting that the risk/benefit ratio for a procedure which compromises the immune system and can lead to serious complications such as Graft versus Host disease or even death.74 BBDF believes that stem cells have tremendous potential. However, a great deal of work remains before stem cell therapy can be safely and effectively applied to defects with transmembrane defects in every cell of our most complex organ where all of the biological cues needed to develop stem cells into the 133 brain cell types have yet to be determined. Based on these and other factors, BBDF does not currently support this avenue of therapeutic development. However, BBDF is tracking advancements that increase the potential to replace CLN3-affected cells with healthy CLN3+/+ brain cells and reduce procedural risks.
Nutrition
In the event of injury or disease, our bodies unleash armies of cellular troops to slaughter invading bacteria, viruses, and clear out the casualties of war on our health and well-being. The increased blood flow, swelling and other activities are all part of the necessary healing process from temporary insults. However, in conditions like CLN3 disease, Alzheimer’s disease and other chronic illnesses of the brain, the sustained activation of the brain’s immune cells is a problem. Not only does it waste energies and immune system reserves; chronic inflammation exacerbates and may accelerate CLN3 and other brain diseases.73,74, 75 But slowing disease isn’t as simple as quelling inflammation. Anti-inflammatory drugs can have dangerous side effects making patients more susceptible to serious infections. Tamping inflammation down too much leaves one unprotected and overly vulnerable to a host of infections. Perhaps anti-inflammatory lifestyle choices can inhibit disease progression without the added danger immunosuppression has to offer.
In 2013, Dr David Perlmutter published the first edition of “Grain Brain: the surprising truth about wheat, carbs and sugar – your brain’s silent killers.”78 More than 1,900 studies were combined to create the Dietary Inflammatory Index published in 2014.78 In 2018, researchers found that eating a Mediterranean diet slows some changes in the brain that may indicate early Alzheimer’s disease.75,76 The MIND diet, a hybrid of the Mediterranean and Dietary Approaches to Stop Hypertension (DASH) diet developed to lower blood pressure, has been shown to slow age-associated cognitive decline.75-77 Interestingly, the MIND diet includes foods rich in L-carnitine, curcumin and vitamin D; supplements studied for their potential to prevent or treat adult forms of neurodegeneration.75-77 Large scale follow-up studies are underway to better understand the potential role of overall diet patterns versus single-agent cells-in-a-dish and animal model investigations. Until then, there is no harm in adding anti-inflammatory ingredients to one’s diet and avoiding pro-inflammatory ingredients. Increase good fats. Avoid carbohydrates, sugar, and gluten.
Nutraceuticals
A great deal of interest has been generated over the last several years regarding the role of nutraceuticals, food particles which render health benefits, to treat neurodegenerative diseases like Multiple Sclerosis, Alzheimer’s Disease (AD) and Parkinson’s Disease (PD). There are no CLN3 or Batten-specific human clinical trials. However, many of the same brain cell abnormalities found in adult brain disease [i.e. inflammation, oxidative stress, protein aggregation] occur in CLN3 disease. Therefore, it stands to reason that vitamins, minerals or other supplements that treat the same brain cell abnormalities and have a positive impact on the health of patients with adult neurodegenerative disease, could have a positive impact on the brain cells, health and overall well-being of children and young adults with CLN3 disease.
The aim of this section is to share a list of nutriceuticals with the Batten community that have been reported to be beneficial in treating illness related to CLN3 disease, in CLN3 cells or model systems or have been called to our attention by families of children and young adults with CLN3 disease. The list is for informational purposes only and is not meant to be or to replace medical advice from a trained physician.
Closing
To support and to accelerate each of the following, BBDF supports multipronged, adaptive and collaborative approaches to disease research and development. By working together with academic researchers, contract research organizations, other Batten and neurodegenerative disease nonprofits, government and the Pharmaceutical industry, In addition to BBDF-specific programs, we seek to keep families, friends and other stakeholders aware of the State of the Science.
References
- Stengel O. Report about a strange illness in four siblings in the vicinity of Røros [in Norwegian]. Hager P, Andersen T, trans. Eyr. 1826;1:347-352
- Batten FE. Cerebral degeneration with symmetrical changes in the maculae in two members of a family. Transactions of the Ophthalmological Societies of the United Kingdom 1903;23:386–90.
- Batten FE. Family cerebral degeneration with macular change (so-called juvenile form of family amaurotic idiocy). Quart. J. Med 1914;7:444-53.
- Mole SE. The genetic spectrum of human neuronal ceroid-lipofuscinoses. Brain Pathol 2004;14:70-6.
- Mole SE, Cotman SL. Genetics of the neuronal ceroid lipofuscinoses (Batten disease). Biochim Biophys Acta 2015;10 PtB:2237-41.
- Benitez BA, Alvarado D, Cai Y, et al. Exome-Sequencing Confirms DNAJC5 Mutations as Cause of Adult Neuronal Ceroid-Lipofuscinosis.
- Berkovic SF, Carpenter S, Andermann F, et al. Kuf’s Disease: a critical reappraisal. Brain 1988;Vol 111, Issue 1:27-62.
- Mitchison HM, O’Rawe AM, Lerner TJ, et al. Refined localization of the batten disease gene (CLN3) by haplogype and linkage disequilibrium mapping to D16S288-D16S383 and exclusion from this region of a variant form of batten disease with granular osmiophilic deposits. Am J Med Genet 1995;57,2:312-15.
- Mole SE, Schulz A, Haltia M. Chapter 4 The neuronal ceroid-lipofuscinoses (Batten disease). Rosenberg’s Molecular and Genetic Basis of Neurological and Psychiatric Disease. Elsevier. 2020.
- Stephen Goldberg, MD. Chapter 1. General Organization. Clinical Neuroanatomy made ridiculously simple. MedMaster, Inc. 2014.
- Onyenwoke RU ad JE Brenman. Lysosomal Storage Diseases – Regulating Neurodegeneration. J Exp Neurosci 2015;9(Suppl 2):81-91.
- Augustine EF, Mink JW. Juvenile NCL (CLN3 Disease): Emerging Disease-Modifying Therapeutic Strategies.Pediatr Endocrinol Rev. 2016;13 Suppl 1:655-662.
- Bosch ME, Aldrich A, Fallet R, et al. Self-Complementary AAV9 Gene Delivery Partially Corrects Pathology Associated with Juvenile Neuronal Ceroid Lipofuscinosis (CLN3).
- Sleat DE, Gedvilait E, Zhang Y, et al. Analysis of large-scale whole exome sequencing data to determine the prevalence of genetically-distinct forms of neuronal ceroid lipofuscinosis. Gene 2016. 593, 284-291.
- Centa JL, Jodelka FM, Hinrich AJ, et al. Therapeutic efficacy of antisense oligonucleotides in mouse models of CLN3 Batten disease. Nat Med 2020;Jul 27.
- S. Food and Drug Administration, Center for Drug Evaluation and Research. Brineura NDA NUMBER approval letter, April 27, 2017. Retrieved August 4, 2020 from www.accessdata.fda.gov/drugsatfda_docs/nda/2017/761052Orig1s000Approv.pdf
- Mirza M, Vainshtein A, DiRonza A, et al. The CLN3 Gene and Protein: What we know. Mol Genet Genomic Med. 2019 Dec;7(12).
- Jafari D, Shajari S, Jafari R, et al. Designer Exosomes: A New Platform for Biotechnology Therapeutics [published online ahead of print, 2020 Aug 4] BioDrugs 2020;10.1007/s40259-020-00434-x. doi:10.1007/s40259-020-00434-x.
- Aberg, L.E., Bäckman, M., Kirveskari, E., and Santavuori, P. (2000). Epilepsy and antiepileptic drug therapy in juvenile neuronal ceroid lipofuscinosis. Epilepsia 41, 1296-1302.
- Pearce DA and F Sherman A yeast model for the study of Batten disease. PNAS 1998 95;(12:)6915-18.
- Pearce DA, Ferea T, Nosel SA, et al. Action of BTN1, the yeast orthologue of the gene mutated in Batten disease. Nat Genet 1999 May;11(1):55-8.
- Gobalek AA, Kida E, Walus M, et al. CLN3 Protein Regulates Lysosomal pH and alters intracellular processing of Alzheimer’s Amyloid-Beta Protein Precursor and Cathepsin D in Human Cells. Mol Genet Metab 2000 Jul;70(3):203-13.
- Holopainen JM, Saarikoski J, Kinnunen PKJ, Jarvela I. Elevated lysosomal pH in neuronal ceroid lipofuscinoses (NCLs) Eur J Biochem 2001 Vol268, Iss22.
- Fossale E, Wolf P, Espinola JA, et al. Membrane trafficking and mitochondrial abnormalities precede subunit c deposition in a cerebellar cell model of juvenile neuronal ceroid lipofuscinosis. BMC Neurosci 2004 5;57
- Luiro K, Yliannala K, Ahtiainen L, et al. Interconnections of CLN3, Hook1, and RAB proteins link Batten disease to defects in the endocytic pathway. Hum Mol Genet 2004 Dec 1;13(23):3017-27.
- Gachet Y, Codlin S, Hyams JS, et al. BTN1, The Schizosaccharomyces Pombe homologue of the human Batten disease gene CLN3, regulates vacuole homeostasis. J Cell Sci 2005 Dec 1;118(Pt 23):5525-36.
- Ramirez-Montealegre D and DA Pearce. Defective lysosomal arginine transport in juvenile Batten disease Hum Mol Genet 2005 Dec 1;14(23):3759-73.
- Cao Y, Espinola JA, Fossale E, et al. Autophagy is disrupted in a knock-in mouse model of juvenile neuronal ceroid lipofuscinosis. J Biol Chem 2006 Jul 21;281(29):20483-93.
- Padilla-Lopez and DA Pearce. Saccharomyces cerevisiae lacking Btn1p modulate vacuolar ATPase activity to regulate pH imbalance in the vacuole J Biol Chem 2006 Apr 14;281(15):10273-80.
- Codlin S, Haines RL, Mole SE. BTN1 Affects endocytosis, polarization of sterol-rich membrane domains and polarized growth in schizosaccharomyces pombe Traffic 2008 Jun;9(6):936-50.
- Metcalf DJ, Calvi AA, Seaman MNj, et al. Loss of the Batten disease gene CLN3 prevents exit from the TGN of the mannose 6-phosphate receptor Traffic 2008 Nov;9(11):1905-14.
- Rusyn E, Mousallem T, Persaud-Sawin D-A, et al. CLN3P impacts galactosylceramide transport, raft morphology, and lipid content. Pediatr Res 2008 Jun;63(6):625-31.
- Codlin S and SE Mole. S. Pombe BTN1, the orthologue of the Batten disease gene CLN3, is required for vacuole protein sorting of CPY1P and golgi exit of VPS10P. J Cell Sci 2009 Apri 15;122(Pt8):1163-73.
- Cotman SL and JF Staropoli. The juvenile Batten disease protein, CLN3, and its role in regulating anterograde and retrograde post-Golgi trafficking. Clinical lipidology 2012. 7; 79-91.
- Kollman K, Uusi-Rauva K, Scifo E, et al. Cell biology and function of neuronal ceroid lipofuscinosis-related proteins Biochim Biophys Acta 2013 Nov;1832(11):1866-81.
- Tecedor L, Stein CS, Schultz ML, et al. CLN3 loss disturbs membrane microdomain properties and protein transport in brain endothelial cells. J Neurosci 2013 33, 18065-18079.
- Kama R, Kanneganti V, Ungermann C, et al. The yeast Batten disease orthologue BTN1 controls endosome-golgi retrograde transport via SNARE assembly J Cell Biol 2011 Oct 17;195(2):203-15.
- Vidal-Donet JM, Carcel-Trullois J, Casanova B, et al. Alterations in ROS activity and lysosomal pH account for distinct patterns of macroautophagy in LINCL and JNCL fibroblasts 2013;8(2);e55526
- Schultz ML, Tecedor L, Stein CS, et al. CLN3-deficient cells display defects in the ARF1-CDC42 pathway and actin-dependent events PLoS One 2014 May 2;9(5):e96647.
- Chandrachud U, Walker MW, Simas AM, et al. Unbiased cell-based screening in a neuronal cell model of Batten disease highlights an interaction between CA2+ homeostasis autophagy, and CLN3 protein function J Biol Chem 2015 Jun5;290(23):14361-80.
- Schmidtke C, Tiede S, Thelen M, et al. Lysosomal proteome analysis reveals that CLN3-defective cells have multiple enzyme deficiencies associated with changes in intracellular trafficking J Biol Chem 2019 Jun 14;294(24):9592-9604.
- Sardiello M, Palmieri M, diRonza A, et al. A gene network regulating lysosomal biogenesis and function Science 2009 Jul 24;325(5939):473-7.
- Settembre C, DiMalta CD, Polito VA, et al. TFEB links autophagy to lysosomal biogenesis Science 2011 Jun 17;332(6036):1429-33.
- Song W, Wang F, Savini M, et al. TFEB regulates lysosomal proteostasis Hum Mol Genet 2013 May 15;22(10):1994-2009.
- Willet R, Martina JA, Zewe JP, et al. TFEB regulates lysosomal positioning by modulating TMEM55B expression and JIP4 recruitment to lysosomes. Nat Commun 2017 Vol 8 Article number 1580.
- Palmieri M, Pal R, Nelvagal HR, et al. MTORC1-independent TFEB activation via AKT inhibition promotes cellular clearance in neurodegenerative storage diseases. Nat Commun 2017 Feb 6;8:14338.
- Palmieri M, Pal R, Nelvagal HR, et al. Corrigendum: MTORC1-independent TFEB activation via AKT inhibition promotes cellular clearance in neurodegenerative storage diseases. Nat Commun 2017 Jun 13;8:15793.
- Ohtake S and YJ Wang. Trehalose: Current Use and Future Applications. J Pharm Sci 2011 Vol 100 Issue 6 P202-53.
- Jain NK and I Roy. Effect of trehalose on protein structure. Protein Science 2009;18:24-36.
- Pineda M, Walterfang M, Patterson MC. Miglustat in Niemann-Pick Disease Type C Patients: A Review. Orphanet J Rare Dis 2018;13:140.
- Singh G and Correa R. Fibrate Medications. In: StatPearls [Internet]. Treasure Island (FL): StatPearls Publishing;2020 Jan 2020 Feb 7.
- Woitowicz S, Strosznaider AK, Jezyna M, et al. The Novel Role of PPAR Alpha in the Brain: Promising Target in Therapy of Alzheimer’s Disease and other Neurodegenerative Disorders. Neurochem Res 2020 May;45(5):972-988.
- Hong M, Song KD, Lee HK, et al. Fibrates inhibit the apoptosis of Batten disease lymphoblast cells via autophagy recovery and regulation of mitochondrial membrane potential. In Vitro Cell Dev Biol Anim 2015 Vol;52:349-55
- Ghosh A, Corbett GT, Gonzalez FJ, et al. Gemfibrozil and fenofibrate, Food and Drug Administration-approved lipid-lowering drugs, up-regulate tripeptidyl-peptidase 1 in brain cells via peroxisome proliferator-activated receptor alpha: implications for late infantile disease therapy. J Biol Chem 2012;287:38922-35.
- Kim K, Kleinman H, Lee H-J, et al. Safety and potential efficacy of gemfibrozil as a supportive treatment for children with late infantile neuronal ceroid lipofuscinosis and other lipid storage diseases. Orphanet J Rare Dis 2017 Jun 17;12(1):113.
- Lojewski X, Staropoli JF, Biswas-Legrand S, et al. Human IPSC models of neuronal ceroid lipofuscinosis capture distinct effects of TPP1 and CLN3 mutations on the endocytic pathway Hum Mol Genet 2014 Apr 15;23(8):2005-2022.
- Orth M and Bellosta S. Cholesterol: It’s regulation and role in Central Nervous System disorders. Cholesterol 2012;2012:292598.
- Kubota K, Nijnuma Y, Kaneko M, et al. Suppressive effects of 4-phenylbutyrate on the aggregation of pael receptors and endoplasmic reticulum stress. J Neurochem 2006 Jun;97(5):1259-68.
- Daruich A, Picard E, Boatright JH, et al. Review: the bile acids urso- and tauroursodeoxycholic acid as neuroprotective therapies in retinal disease. Mol Vis 2019 Oct 14;25:610-24.
- Ono K, Ikemoto M, Kawarabayashi T, et al. A chemical chaperone, Sodium 4-Phenylbutyric acid, attenuates the pathogenic potency in human alpha-synuclein A30P + A53T transgenic mice. Parkinsonism Relat Disord 2009 Nov;15(9):649-54.
- Wiley JC, Pettan-Brewer C, Ladiges WC. Phenylbutyric acid reduces amyloid plaques and rescues cognitive behavior in AD transgenic mice. Aging Cell 2011 Jun;10(3):418-28.
- Nunes AF, Amaral JD, Lo AC, et al. TUDCA, a bile acid, attenuates amyloid precursor protein processing and amyloid-B deposition in APP/PS1 mice Mol Neurobiol 2012 Jun;45(3):440-54.
- Sturm E, Fellner L, Krismer F, et al. Neuroprotection by epigenetic modulation in a transgenic model of multiple system atrophy Neurotherapeutics 2016 Oct;13(4):871-79.
- Ackerman HD and GS Gerhard. Bile acids in neurodegenerative disorders. Fron Aging Neurosci 2016 Nov;8:263.
- Aldrich A, Bosch ME, Fallet R, et al. Efficacy of phosphodiesterase-4 inhibitors in juvenile Batten disease (CLN3). Ann Neurol 2016 Dec;80(6):909-23.
- Dhar S, Bitting RL, Rylova SN, et al. Flupirtine blocks apoptosis in batten patient lymphoblasts and in human postmitotic-CLN3- and CLN2-deficient neurons. Ann Neurol 2002 Apr;51(4):448-66.
- Maalouf K, Makoukji J, Saab S, et al. Exogenous Flupirtine as Potential Treatment for CLN3 Disease. Cells. 2020. Aug 11;9(8):E1872.
- Cialone J, Augustine EF, Newhouse N, et al. Parent-reported benefits of flupirtine in juvenile neuronal ceroid lipofuscinosis (Batten disease;CLN3) are not supported by quantitative data. J Inherit Metab Dis 2011 Oct;34(5):1075-81.
- Kim T, Vidal GS, Diurisic M, et al. Human LILRB2 is a beta-amyloid receptor and its murine homolog PIRB regulates synaptic plasticity in an Alzheimer’s model. Science 2013 Sep 20;341(6152):1399-404.
- Chattopadhyay S, Kriscenski -Perry E, Wenger DA, et al. An autoantibody to GAD65 in sera of patients with juvenile neuronal ceroid lipofuscinosis. Neurology 2002 Dec 10;59(11):1816-7.
- Seehafer SS, Ramirez-Montealegre D, Wong AM, et al. Immunosuppression alters disease severity in juvenile Batten disease mice. J Neuroimmunol 2010;230:169–172.
- Augustine EF, Beck CA, Adams HR, et al. Short-term administration of mycophenolate is well-tolerated in CLN3 disease (juvenile neuronal ceroid lipofuscinosis). JIMD Rep 2019;43:117-124.
- Groh J, Berve K, Martini R. Fingolimod and teriflumide attenuate neurodegeneration in mouse models of neuronal ceroid lipofuscinosis. Mol Ther 2017;25:1889-99.
- Brondon JE, Page K, Polishchuck V, et al. Outcomes of Umbilical Cord Blood Transplantation in Children with Batten disease. Abstracts/Biol Blood Marrow Transplant 2019 462 Vol 25 Iss3 Supplement.
- Morris MC, Tangney CC, Wang Y, et al. MIND diet associated with reduced incidence of Alzheimer’s disease. Alzheimers Dement. 2015. Sep;11(9):1007-14.
- Morris MC, Tangney CC, Wang Y, et al. MIND diet slows cognitive decline with aging. Alzheimers Dement. 2015 Sep;11(9):1015-22.
- Morris, Martha Clare. Diety for the MIND: The Latest Science on What to Eat to Prevent Alzheimer’s and Cognitive Decline. New York City. Hachette Book Group. 2017.
- Perlmutter D and Loberg K. Grain Brain. New York City. Hachette Book Group. 2013.
- ShivappaN, Steck SE, Hurley TG, et al. Designing and Developing a literature-derived, population-based dietary inflammatory index. Public Health Nutr. 2014. Aug;17(8):1689-96.